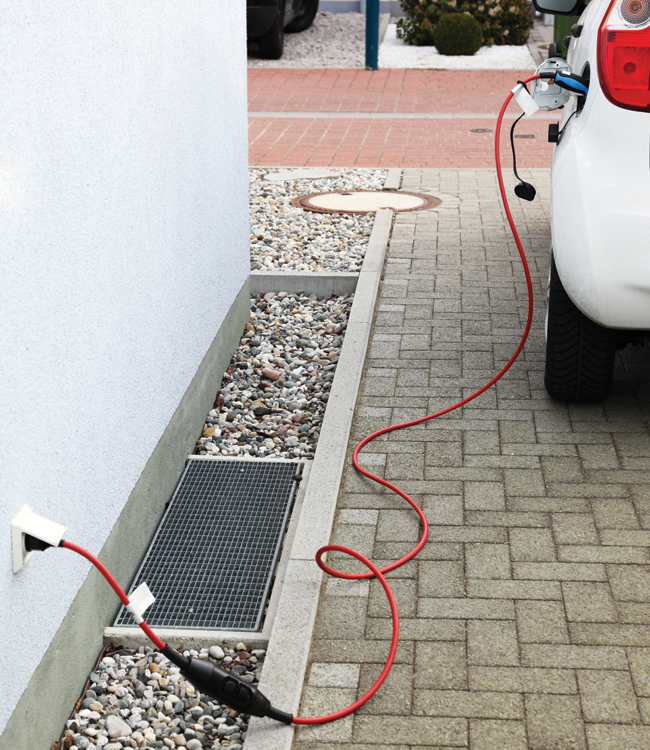
Since the early 20th century, AC has been the common source of supply and load within buildings, although there have always been elements of DC load, such as fire-alarm systems and emergency lighting. In recent years, there has been an increase in demand for other elements that require a DC supply – such as LED lighting and, on the generation side, photovoltaic panels – and there are more on the horizon, including fuel cells (generation) and electric cars (supply). This increase has been recognised by the IET with the publication of a Code of Practice on DC distribution in buildings.
With digital devices such as mobile phones and laptops all requiring DC power, the ‘base’ electrical load in most new homes is now mainly from DC loads. DC fan coil units – used for space heating and cooling – are typically 50% more efficient than AC versions; they offer seamless change in fan speed and operate on a 0-10V DC supply. Mechanical ventilation with heat recovery (MVHR) units also generally use DC fan motors with 0-10V input (see panel, ‘Typical voltages’).
Typical Voltages
Mobile phones and tablets (5V)
TV and PC screens (24V)
PCs (typically 500 to 900 watts rating with 100V to 240V AC in, 12V DC out)
Motors (MVHR, fan coil unit, typical 0-10V)
Electric vehicles (330V for Mitsubishi i-MiEV; 360V for BMWi3 and Renault Zoe)
The motor outputs on fan coil units and MVHR need to vary to control the speed of the fan motor. With AC, the variable voltage input to the motor is achieved by adding a resistor in the supply circuit, so electricity use at full and low speed is very similar. With a DC motor, the AC/DC or DC/DC voltage conversion can vary the voltage to the DC motor to control the fan speed – so, halve the speed and you roughly halve the electricity use.
DC generation and storage
PVs have been around for a considerable time generating DC power, but only during the day, with their output dependent on the solar density. In the UK, this results in a typical load factor (percentage of continuous rated output against actual output for a typical year) of about 8%. This does not preclude the use of PVs as a source of electricity generation if there is battery storage, but it does impact on their economic viability.
Many DC loads in buildings do now match the period when PVs may be at peak output; a battery can then be charged, and discharged later to supply the DC load. However, the cost of a battery in addition to PVs makes the system more expensive than grid electricity.
Fuel cells that generate electricity output at DC are a potential game-changer. Research spending on this technology has increased greatly in the US and Japan over the past few years – with a view to developing electric cars – and this has driven down the cost of generating power in this way. According to the US Department of Energy, the price has gone from $124/kW in 2006 to $55/kW in 2014, with a target of $40/kW by 2020 and $30/kW in the longer term.
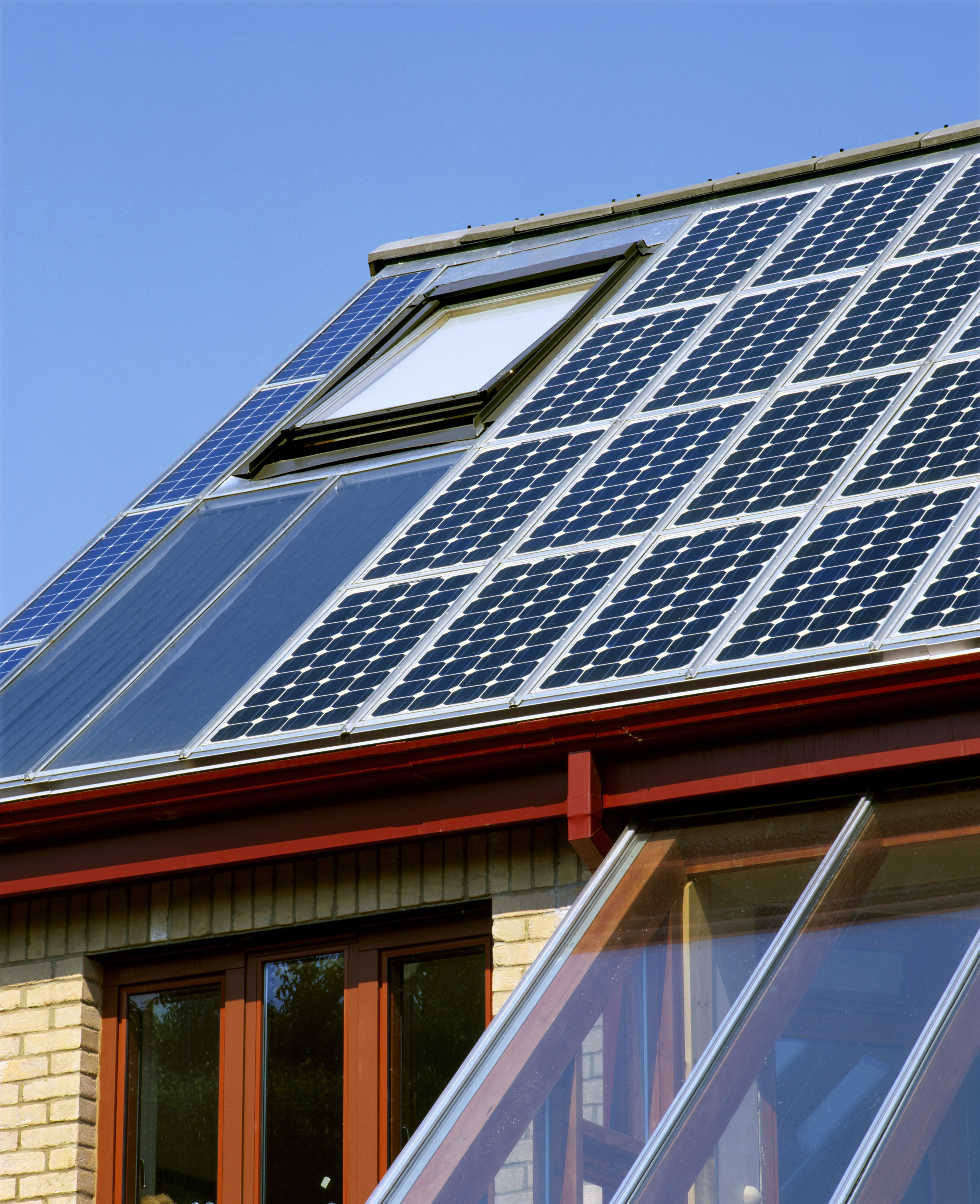
Sue Roaf's home in Oxford generates electricity that either feeds into the national grid or charges the batteries on her electric car. Roaf is professor of architectural engineering at Heriot-Watt University
Credit PHOTOFUSION/ GETTY IMAGES
There are several types of PVs and these are arranged with individual cells configured into arrays, which are then joined together to produce the output voltage at the point of connection. This can be up to 600V (before inversion) for large PV sites with multiple arrays. Fuel cells broadly fall into two camps: high-temperature cells (200°C to 1,100°C) giving greater power output, typically 100MW or more; and low-temperature cells (20°C to 100°C), with outputs of 100kW or less.
The low-temperature fuel cells can be configured to give a set voltage output with a constant input to the fuel cell. By controlling the input – ie storing the gas – you can control the electricity output to suit demand.
A number of storage options are available, the most common being ‘chemical conversion’, such as in static batteries or fuel cells. As these are both DC, they can accept direct connection from PV arrays or store the gas input to the fuel cell, where the gas acts as the storage medium.
A fuel cell gives output at the same time as it receives the gas input, which can be stored so you can time the output to suit the load.
By removing the need to convert from AC to DC, several stages in the voltage-conversion process are removed, thereby increasing the conversion efficiency
Voltage conversion
An AC transmission and distribution network is in place, with standards harmonised across Europe at 230V (single phase) and 400V (three phase). All electrical equipment is currently designed to suit an AC connection, with DC loads connected mainly via a switched mode power supply unit, which converts AC to the required DC voltage. These power supply units are often less than 80% efficient, although several bodies offer approval schemes to encourage improved efficiency.
By removing the need to convert from AC to DC, several stages in the voltage-conversion process are removed, thereby increasing the conversion efficiency – from an efficient AC/DC voltage conversion of 85% to closer to 90% with DC/DC conversion. The need to change between the generated and required voltage of the DC load is unlikely to be removed in the near future, because generating voltages are significantly higher than most commercial or domestic DC load items – with the potential exception of electric-car charging.
DC in the home
Given the efficiency gain in DC-to-DC step-up or step-down voltage conversion – where DC generation is located close to the served DC load – there is a benefit to DC distribution between the PVs or fuel cell and the DC load being supplied; as would be the case with the storage of DC-generated electricity with batteries. A study – part- funded by the Department of Trade and Industry, in collaboration with Moixa Energy – was carried out into the use of battery storage to supply DC lighting and 5V DC USB outlets (for portable devices such as tablets and phones) in a typical residential dwelling.
Figure 1 adds a PV array to the scenario. The battery allows the variable output of the PV to balance with the various DC loads within the apartment, with direct DC/DC conversion from a typical 50V PV array output to 12V for the LED lighting and 5V for the USB circuit. With the MVHR also connected to the system the whole house is lit and ventilated using power generated by the PV.
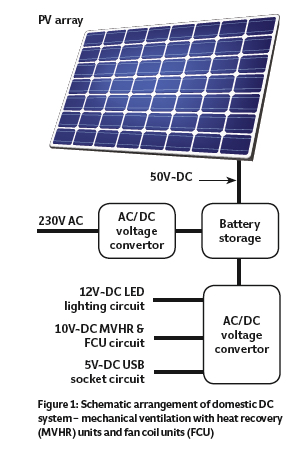
There are two significant changes on the horizon – electric-car charging and fuel cells. Currently a typical electric car – based on a Renault Zoe, four-door family car – needs 22kWh of charge for a 100-mile journey, which requires either a rapid one-hour charge (61A at 360V DC) or, more typically, a longer seven-hour charge (8.7A at 360V DC).
For one house, 92m2 of PV (8m2 per 1kW peak with a capacity factor of 8%) would be needed for a full 100-mile car recharge each day, over seven hours, with battery storage to align the PV output to when the charging is required – most probably overnight. The fuel-cell voltage is more likely to be matched to the electric car and would be more viable in terms of the space needed in the home.
Conclusions
PV costs have dropped significantly in a very short space of time, with the Solar Association claiming a reduction in price, including installation, of 60% in the past five years.
While the AC supply to buildings from the national transmission and distribution network is unlikely to change any time soon, there will be benefits for buildings with DC generation from PV arrays and fuel cells because of the conversion efficiency of DC to DC and DC distribution within buildings.